
New materials and compounds driving sustainable chemistry innovation
Biomass feedstocks, biopolymers, biopolymer composites and more
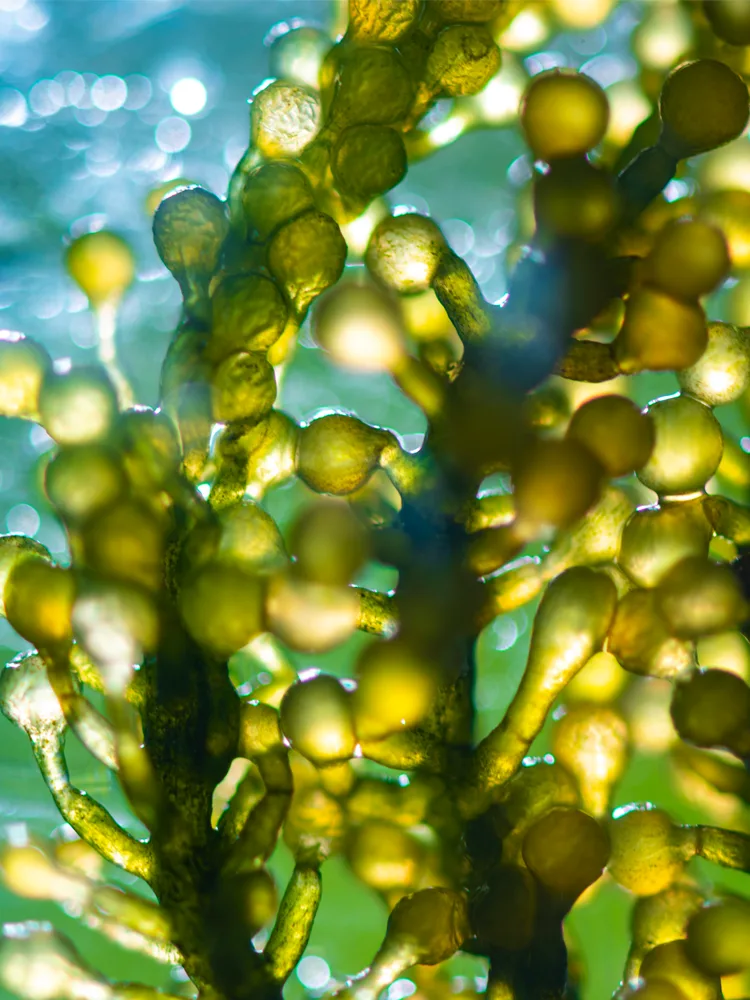
Net zero and sustainable development goals have compelled many industries to discover and develop new materials for their products. To achieve this transition, engineers, researchers, product developers and other industry professionals are tasked with influencing and updating systems that have been in place for decades or longer. Innovation has become a greater necessity in the chemicals industry, driving the development of novel solutions in biomass feedstocks, biopolymers, biopolymer composites and more.
Sustainable Feedstocks
Photosynthetic organisms as sustainable feedstocks
Photosynthetic organisms like microalgae 新しいタブ/ウィンドウで開く and cyanobacteria are seen as carbon-neutral, sustainable feedstocks as their cultivation does not require arable land, pesticides and agricultural machinery. Brown algae 新しいタブ/ウィンドウで開く, for instance, has a dry weight composed of 40% alginates, which can be blended with starches to create bioplastics. These organisms' photosynthetic machinery also makes them an ideal source of biomass feedstocks for biofuel. Additionally, cyanobacterial strains have been used for producing ethanol 新しいタブ/ウィンドウで開く, isobutanol 新しいタブ/ウィンドウで開く (a potential gasoline substitute), ethylene 新しいタブ/ウィンドウで開く and isoprene 新しいタブ/ウィンドウで開く.
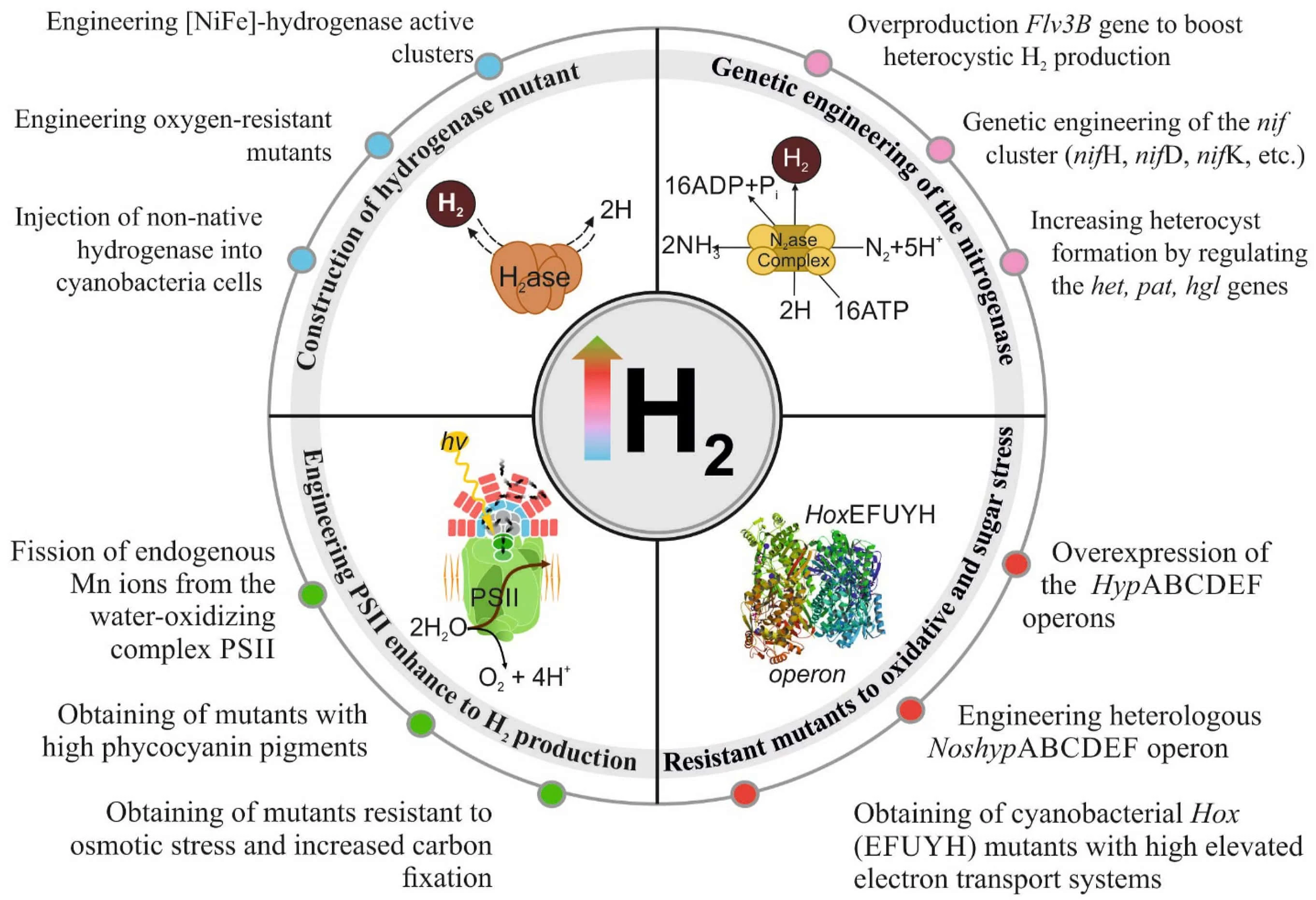
Pathways to increase H2 cleavage in cyanobacterial strains (Source: International Journal of Hydrogen Energy 新しいタブ/ウィンドウで開く)
Researchers have proposed the following methods for connecting these biomass feedstocks to existing production processes:
Conversion to methane 新しいタブ/ウィンドウで開く through fermentation
Release of the biomass’s biomolecular components 新しいタブ/ウィンドウで開く (e.g., carbohydrates, cellulose, lipids, nucleic acids, proteins) through biorefinery methods, such as mechanical cell cracking, hydrothermal liquefaction and anaerobic digestion 新しいタブ/ウィンドウで開く
The production of hydrogen via genetically engineered cynobacteria 新しいタブ/ウィンドウで開く
While the global biofuel market is projected to reach $225.9 billion by 2028 新しいタブ/ウィンドウで開く, for instance, there remain obstacles toward large-scale adoption of these products, including high production cost and the need for more matured technology. 新しいタブ/ウィンドウで開く
Residual biomass as a circular resource
Waste as a source of biomass feedstocks supports circularity goals. It’s also an ideal resource for confronting the problems inherent in first-generation feedstocks, such as the negative environmental impact of cutting down trees or planting water-hungry crops for fuel and plastic production.
Resources for residual biomass include:
Food industry waste — e.g., fruit seeds, root-crop peels, crustacean shells
Agricultural waste — e.g., corn cobs, rice husks, sugarcane bagasse, wheat bran
Biowaste from effluents — e.g., household wastewater, oil industry effluents
Other types of waste — e.g., paper, municipal solid waste (household trash)
A project by Repsol aims to use residual soft-wood and plant waste as biofuel 新しいタブ/ウィンドウで開く feedstocks through hydrolysis. The proposed biorefinery could lower CO2 emissions by 510,000 tons a year if successful. Triglyceride-rich waste oils such as vegetable oil can be transformed into epoxy resins 新しいタブ/ウィンドウで開く through epoxidation, while limonene (an essential oil in citrus peels) can be used as a feedstock for bio-based polycarbonate 新しいタブ/ウィンドウで開く to create a sustainable plastic resin.
Biopolymers
Biopolymers are widely used in food processing, drug delivery, and regenerative medicine. They’re particularly promising materials in the search for sustainable alternatives 新しいタブ/ウィンドウで開く for plastic films, packaging and electronic and medical components.
But with the push toward net zero goals, the industry has been reckoning with the fact that not all biopolymers are sustainable. In fact, roughly half of the bioplastics 新しいタブ/ウィンドウで開く in the market are non-biodegradable. Biopolymers (and bioplastics, by extension) may be bio-based but non-biodegradable, fossil-based but biodegradable, or bio-based and biodegradable.
Bio-based but non-biodegradable polymers are not necessarily more sustainable if their farming and production processes result in a significant carbon footprint. One cradle-to-grave life cycle assessment 新しいタブ/ウィンドウで開く found that bio-based polyethylene terephthalate (PET) bottles may have a worse environmental impact than fossil-based ones.
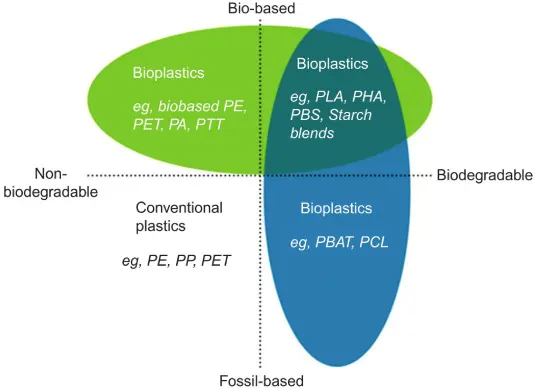
Classification of bioplastics (Source: Recycling of bioplastic waste: A review 新しいタブ/ウィンドウで開く)
Further complicating the situation, fossil-based but biodegradable polymers also fall under the umbrella term “biopolymers.” Polybutylene adipate-co-terephthalate (PBAT) is derived from organic compounds (1,4-butanediol and adipic acid and the polymer of dimethyl terephthalate) and is non-renewable. It is also fully biodegradable. In a home compost, PBAT can degrade within three to six months 新しいタブ/ウィンドウで開く.
As a co-polyester 新しいタブ/ウィンドウで開く, PBAT combines the biodegradability of aliphatic polyesters with the mechanical properties of aromatic ones. It shares the stretchable, film-like property of low-density polyethylene (PE), making it a popular material for products like compostable trash bags 新しいタブ/ウィンドウで開く (when combined with polylactic acid [PLA]) and degradable mulch films for agriculture.
However, in soil, PBAT microplastic (PBAT-MP) degradation 新しいタブ/ウィンドウで開く may produce chemicals that can be highly toxic to plants. PBAT-MPs in the soil may also “severely disrupt” the photosynthetic system of Arabidopsis (also known as thale cress), a model organism for studying plant biology, genetics and pathology.
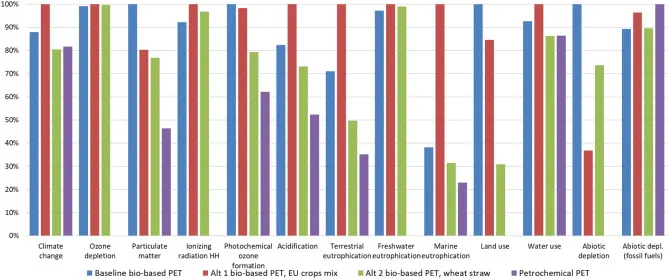
Comparison of the cradle-to-grave impact of bio-based and petrochemical PET bottles per functional unit. (Source: Science of The Total Environment 新しいタブ/ウィンドウで開く)
Even biopolymers that are both biomass-derived and biodegradable may not be truly sustainable when scrutinized from a life-cycle perspective due to carbon-intensive cultivation and manufacturing processes. Additives like pigments, flame retardants, lubricants and thermal stabilizers are often environmentally harmful 新しいタブ/ウィンドウで開く as well.
The term "biodegradable" can also be misleading as it does not necessarily mean compostable. Consider PLA, a polymer based on sugarcane or cornstarch that is used for surgical sutures. PLA production emits 68% fewer greenhouse gasses 新しいタブ/ウィンドウで開く compared to traditional plastic production. But PLA also takes 100 to 1,000 years 新しいタブ/ウィンドウで開く to decompose in a landfill — a process shortened to months only with the use of industrial composters running at high temperatures in specific conditions.
Given these problems and evidence of widespread greenwashing, the industry needs to accelerate innovation to produce truly sustainable polymer products.
Waste as a source of sustainable biopolymers
Novel biodegradable polymers derived from biomass waste 新しいタブ/ウィンドウで開く are emerging as more sustainable materials that promote a circular ecosystem. Experiments abound: Starch-based bioplastics derived from potato peels 新しいタブ/ウィンドウで開く have been found to degrade at a similar rate as paper. Pectin and cellulose from citrus peels 新しいタブ/ウィンドウで開く and various rinds (watermelon, cantaloupe, pineapple) can be used to create a bioplastic film suitable for food packaging.
Another novel basis for sustainable bioplastic film is the single-cell protein 新しいタブ/ウィンドウで開く (SCP) extracted from dried biomass like algae, bacteria and fungi found in agricultural waste and industrial byproducts. This makes SCPs an attractive alternative protein source for first-generation feedstocks like soybean, milk casein, pea and cottonseed protein.
Sustainable substrates for producing polyhydroxybutyrate (PHB), a 100% biodegradable polymer, can be derived from dairy and agro-industrial waste 新しいタブ/ウィンドウで開く, as well as from active sludge from wastewater treatment 新しいタブ/ウィンドウで開く in the food processing industry. PHB’s mechanical and thermoplastic properties are comparable with those of polypropylene (PP) and PE, making it a responsible alternative material for packaging, beverage containers, disposable utensils and coatings. As it is biocompatible, PHB is also used for medical implants and drug delivery carriers.
Biopolymer composites
While commonly used for plastics, biopolymer composites are seeing increased interest from the energy sector 新しいタブ/ウィンドウで開く. Their biocompatibility and biodegradability allow biopolymers to enhance the performance of other biologically active molecules in electrochemical devices. For example, a high-conducting biopolymer membrane 新しいタブ/ウィンドウで開く for a lithium-ion conducting battery can be made by adding lithium chloride salt to tamarind seed polysaccharide.
In general, biopolymers tend to have poorer mechanical properties than their traditional counterparts, they need to be reinforced 新しいタブ/ウィンドウで開く with fillers or nanofillers, which may often be fossil-based.
Attempts to make biopolymer composites more sustainable take different approaches. One method is to make the conventional additive or filler more eco-friendly by changing its source or production methods. Another is to identify bio-based alternatives that can effectively reinforce biopolymers.

Biopolymer composites as energy storage materials (Source: Journal of Environmental Chemical Engineering 新しいタブ/ウィンドウで開く)
Reducing the carbon footprint of fossil-based fillers
Sustainability is not simply a question of materials. Carbon emissions can also be reduced by redesigning processes and equipment, especially given the difficulty of changing entire systems or factories to produce a new material.
Some manufacturers reduce their reliance on petrochemical polymers by using them as fillers instead of as base polymers, as well as by using reclaimed materials. Algopack, a French biotech company founded in 2010, sells plastic granules based on algae blended with PP and PE 新しいタブ/ウィンドウで開く. Green Dot Bioplastic’s Terratek WC product line 新しいタブ/ウィンドウで開く comprises composites of wood fibers from lumber yard waste and reclaimed PP. These composites are used for furniture, decking, fences and children’s toys. Although PP is a non-biodegradable petrochemical polymer, using reclaimed and recycled PP has been found to reduce its carbon footprint by 42.8% compared to using virgin PP 新しいタブ/ウィンドウで開く.
Investigating biomass sources for effective fillers
Still, researchers are finding that biomass sources can have mechanical and thermal properties to match that of petrochemical fillers. One study found essential oils, such as those from bergamot, clove, lemongrass and rosemary, to be effective bio-based plasticizers for PLA 新しいタブ/ウィンドウで開く. These oils imparted antibacterial properties to PLA-based bactericidal film, which is used in horticulture. A separate study combined cellulose nanofibers (CNF) isolated from plant biomass with zinc oxide (ZnO) to create a nanocomposite for reinforcing natural rubber film 新しいタブ/ウィンドウで開く. The resulting composite (CNF-ZnO with natural rubber) showed a 42% improvement in tensile strength compared to regular natural rubber film.
Waste-derived biomass enhances the sustainability of filler alternatives. When researchers added biochar derived from wood and sewage sludge 新しいタブ/ウィンドウで開く to PLA and Bioplast GS 2189, they found that the waste-derived fillers had an impact on the composites’ mechanical, thermal and optical properties. The biochar-added composites also displayed higher water absorption and rigidity. The resulting biocomposite material can be used to make compostable agricultural accessories like plant supports.
Learn more about sustainable innovation with Elsevier
Read more insights into chemical industry innovation and learn more about how Elsevier supports the world's leading engineering organizations.
エルゼビアの化学業界における持続可能な製造ガイド
